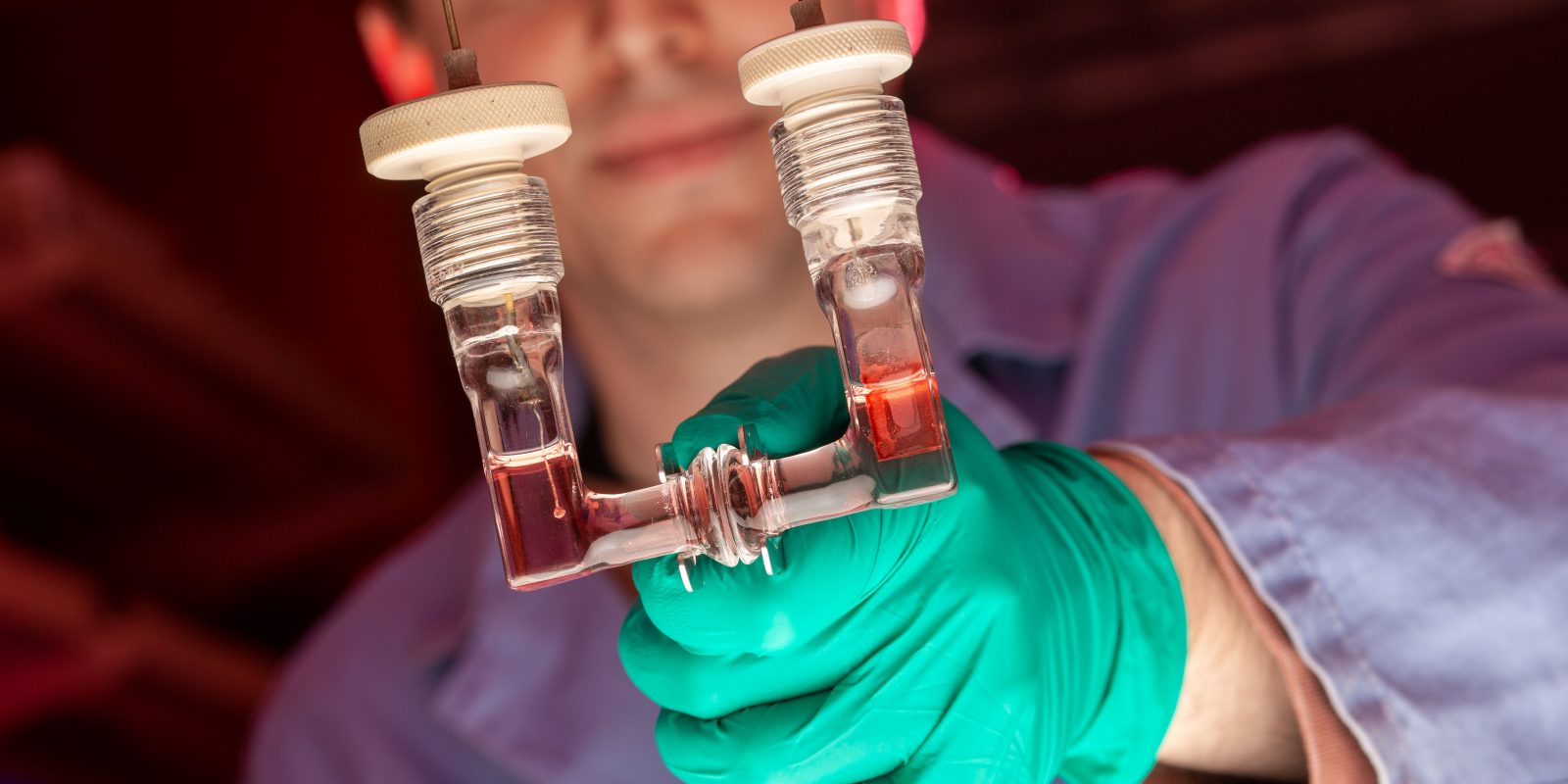
Photo by Mark Graham
Ben Sherman Ties Photosynthesis to Affordable Solar Energy Cells
The chemist is working to improve photovoltaic cells that can convert sunlight.
Through photosynthesis, plants do something simple yet remarkable: They convert sunlight to energy by breaking apart water molecules. If scientists could replicate what plants do every day, the world would have a clean, endless alternative to fossil fuels.
Ben Sherman, assistant professor of chemistry in TCU’s College of Science & Engineering, develops solar cells that are inspired by plants’ energy conversion process. But for human energy needs, he said, the work isn’t so simple. “Photosynthesis is very convoluted … yet somehow this is the very basis of life.”
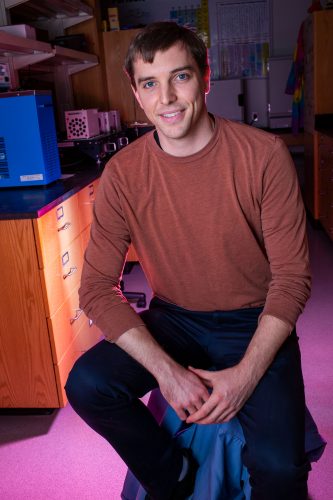
Ben Sherman, assistant professor of chemistry, focuses his research on the electrochemical aspects of creating highly efficient solar cells. Photo by Mark Graham
According to Sherman, the overall efficiency of converting sunlight into chemical energy during photosynthesis is negligible at best. Plants convert only about 1 percent of the sunlight they receive into carbon dioxide products. For a solar cell to compete with fossil fuels, it would need to achieve at least a 10 percent solar-to-fuel efficiency.
Sherman’s research involves assembling and optimizing photovoltaic cells. These devices are similar to batteries, but they produce electricity when sunlight strikes a special material that causes a chemical reaction to occur. His cells are inspired by photosystem II, a protein complex that drives photosynthesis by using the energy from sunlight and a metal-based catalyst to break apart a water molecule into hydrogen and oxygen.
“The objective has always been to mimic that process,” Sherman said. But breaking apart a water molecule, which is stable by nature, requires a potent catalyst.
One area of his research is searching for an inexpensive metal-based catalyst powerful enough to split a water molecule into its component parts. “The best catalysts we can come up with are using elements you don’t find in nature, at least [not] in photosystem II,” he said.
Sherman’s group, which includes graduate students, an undergraduate research student and a postdoctoral researcher, begins with catalysts proven to be capable of breaking apart water. But these catalysts contain iridium or ruthenium, expensive rare-earth metals. His group builds the rest of the photoelectrode using these catalysts, making sure to optimize all of the other parts. The last piece of the puzzle is changing out the catalyst for experimental ones made from cheaper, more abundant metals.
The right catalyst is just one piece of the puzzle. The kind of photovoltaic cells that Sherman’s group studies are called dye-sensitized solar cells, or Grätzel cells, after the Swedish scientist who invented them. Grätzel cells have four basic parts: a transparent glass surface that conducts electricity, a layer of porous semiconductor material coated with a layer of molecular dye that serves as an electrode, the electrolyte solution where the chemistry takes place and a counter electrode.
The dye sits on the surface of the semiconductor material. Reactions that require a catalyst — like splitting water — occur in that solution. These reactions always involve electrons moving from the semiconductor electrode to the counter electrode, creating an electrical current.
“The largest issue facing humanity is climate change. I wanted to apply myself to that issue and that problem to contribute any way I could.”
Ben Sherman
The end goal is to develop a photoelectrochemical system that is feasible on a large scale. Sherman said some existing systems are 18 percent efficient at converting sunlight to fuel, but they typically use costly semiconductors and expensive metal catalysts. “It’s tens of thousands of dollars for a square centimeter of an actual device. So even at that efficiency, it can’t compete [with fossil fuels].”
Optimizing each part of a complicated cell requires a team effort. “Collaboration is so essential,” Sherman said. “I’m doing the writing and getting the grants, but it’s the grad students that do the work, and without them involved, it would be nothing.”
In 2020, Sherman worked with two graduate students, Debora Beeri and Jackson Roye, and an undergraduate student, Maggie Purvis.
Beeri said she pursued PhD work in Sherman’s lab because she wanted to help find clean energy sources. “It’s great that the scientific world is trying to find cures for cancer and other health problems,” she said Sherman told her. “But who is going to heal the Earth that we live in?”
Beeri’s work involves optimizing the glass surface that conducts electricity, and she is investigating new types of semiconductor electrodes using less expensive materials that could eventually be used on a large scale.
Sherman said he is also interested in developing a rechargeable solar battery. “The largest issue facing humanity is climate change. I wanted to apply myself to that issue and that problem to contribute any way I could.”
Your comments are welcome
1 Comment
Is there any use to thinking the surface area is not a problem. If only 1% efficient but covers a LIT of space, some useful quantity of electricity could be had.
Think about the roof of Cowboys stadium and Rangess stadium. At use or non-use times there is a LOT of solar real estate. With such, the 10-20% yield may not be necessary if 19% is saved on the hardware and space for installation becomes easily available.
Installing where large electric feeds are already present for incoming electric could also help from needing to create infrastructure all the way out to the panhandle, beyond the reality of the usage area. Also saves on inefficiencies of long distance transmission. At least till superconductors come along. But those also would be needed for just short distances for instance the mentioned stadiums even up to DFW airport or a Google data storage block with way lots of energy used, could use a shorter distance superconductor like they nitrogen blanket the telephone lead shrouded 1000 pair mainline copper conductor traditional POTS lines underground. Fir just 30 miles instead of hundreds, it could be leveraged for usefulness.
Think of acetobacter reaction to give sugar fermentation to CO2 and ethanol. Sugar plus lactobacillus can give lactic acid and acetic and malic dehydrogenase giving off CO2 as well. The CO2 could be harnessed and reverted each way. A curious reaction is the 6 sugar to pentode sugar pathway, that can run bidirectionally if given the right drivers. Also the electron transfer in cells that can recharge with NAD, FAD, with citric acid regenerative pathway and private as a reverse pathway?
Maybe not as stable in glass doping pigment active form, or May oxidize to lose stability, like caramelizing sugars?
Just thinking the composite project constraints of electric per square cm may pale if the size is so much cheaper, that efficiency doesn’t matter a much if very cheap for large areas easily available.
Still only daylight exciter hours, if it could run off other inputs like being laid along high tension lines in easements already existing, maybe magnetic or ionic charge could have something run even at night (heating overload tone up north) using wasted airborn charge distribution, like a motor winding, not always winding, but linear snatching alternating energy coming off “current” lines already existing. Underneath or halfway down the Aframe high voltage distribution lines. Just sap wasted energy that some are concerned about, homes, schools, etc along 100’ rights of way already set to use energy transmission and like cell units scabbed on may be of some use to “ionize?” the reaction.
Assuming laser excitation would be net negative energy loss, unless helped boost efficiency over a base threshold needed to get to energy creation. Thinking a single laser at photosynthesis 2 wavelength may be great as in photo driven greenhouses with specific wavelengths not losing the broad spectrum of full visual light, but specifically activating the photo 1 and 2 wavelengths. Rather than wasting trying to get incandescent or fluorescent with creating irrelevant wavelengths at cost for non used wavelengths.
Related reading:
Research + Discovery
Climate Change? Have Another Cup of Coffee
Omar Harvey studies novel ways to capture and store carbon dioxide.
Features, Research + Discovery
Failure on a Small Scale: Tiny Holes Elude Silicon Researchers
Professor Jeffery Coffer doesn’t allow frustration to get in the way of solving a riddle.
Features, Research + Discovery
Kristof Pota Manipulates Metals to Treat Alzheimer’s
The chemist also uses metals to better the contrast agent for MRIs.